Moms’ voices get big reactions in kids’ brains
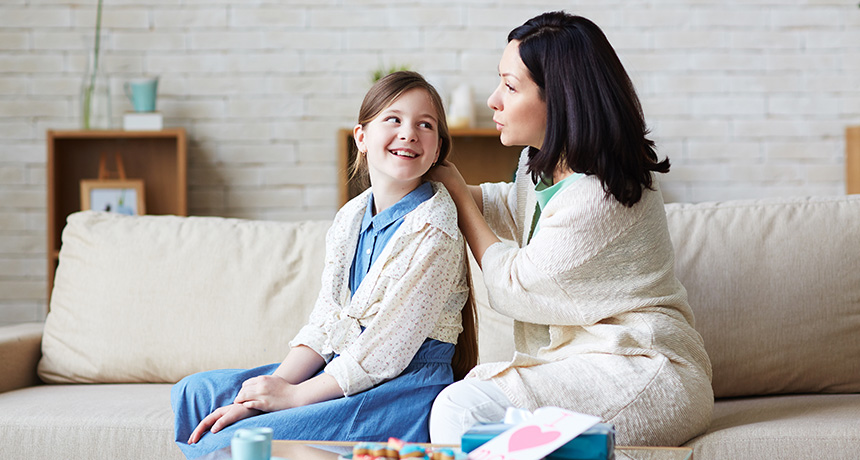
Any parent trying to hustle a school-bound kid out the door in the morning knows that her child’s skull possesses a strange and powerful form of black magic: It can repel parents’ voices. Important messages like “find your shoes” bounce off the impenetrable fortress and drift unheeded to the floor.
But when this perplexing force field is off, it turns out that mothers’ voices actually have profound effects on kids. Children’s brains practically buzz when they hear their moms’ voices, scientists report in the May 31 Proceedings of the National Academy of Sciences. (Fun and not surprising side note: Babies’ voices get into moms’ brains, too.)
The parts of kids’ brains that handle emotions, face recognition and reward were prodded into action by mothers’ voices, brain scans of 24 children ages 7 to 12 revealed. And words were not required to get this big reaction. In the study, children listened to nonsense words said by either their mother or one of two unfamiliar women. Even when the words were fake, mothers’ voices still prompted lots of neural action.
The study was done in older kids, but children are known to tune into their mothers’ voices early. Really early, in fact. One study found that fetuses’ heart rates change when they hear their moms read a story. For a fetus crammed into a dark, muffled cabin, voices may take on outsized importance.
And voices carry particularly powerful messages throughout childhood. “A tremendous amount of emotional information is conveyed to children through auditory channels,” says University of Wisconsin-Madison child psychologist Seth Pollak. And, he points out, kids are small. “Kids’ faces are down around our knees. And children who are crawling are looking at the ground,” he says. This obvious point means that facial expressions and other visual signals might not pack as much punch as a voice.
Of course, voices other than those belonging to moms are also important. Pollak says that voices of fathers — or any other caregiver who spends lots of time around a child — probably affect children’s brains in a similar way. It’s just that those studies haven’t been done yet.
The results of the latest brain scan study make a lot of sense, says Pollak. Some of the brain regions activated are those involved in feeling good. “A caregiver’s voice is actually rewarding. It activates the systems that make us feel calm,” he says.
And the new study might help explain some of Pollak’s earlier results. He and his colleagues stressed out 68 girls, who happened to be the same ages as those in the brain scanning study, by making them do math and word problems in front of three unsmiling adult strangers — a terrifying prospect for most kids. (And adults.) After their ordeal, the girls either talked to their moms in person, on the phone or by instant messenger.
Compared with the instant messenger typers, the girls who saw their moms in person or talked to them on the phone were more soothed, showing lower levels of stress hormones. That finding, published in 2012 in Evolution and Human Behavior, suggests that to a kid, there’s something especially calming about hearing her own mother’s voice.
And now, by showing the widespread reaction to a mother’s voice, the brain data back that up. “It all kind of hangs together in a way that I think is very intuitive,” Pollak says. In other words, a mother’s voice is powerful, perhaps even strong enough to overcome a force field.