A coral pollution study unexpectedly helped explain Hurricane Maria’s fury
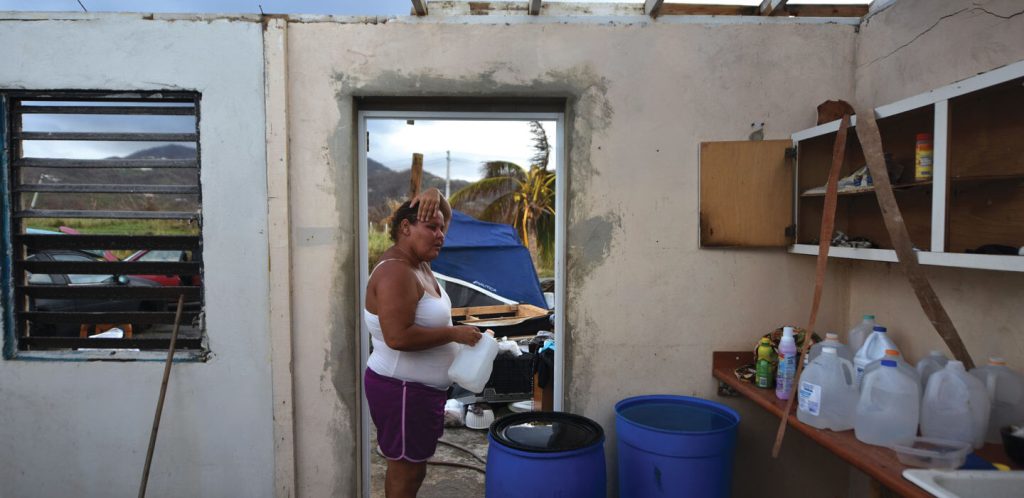
Hurricane Maria struck the island of Puerto Rico early on September 20, 2017, with 250-kilometer-per-hour winds, torrential rains and a storm surge up to three meters high. In its wake: nearly 3,000 people dead, an almost yearlong power outage and over $90 billion in damages to homes, businesses and essential infrastructure, including roads and bridges.
Geologist and diver Milton Carlo took shelter at his house in Cabo Rojo on the southwest corner of the island with his wife, daughter and infant grandson. He watched the raging winds of the Category 4 hurricane lift his neighbor’s SUV into the air, and remembers those hours as some of the worst of his life.
For weeks, the rest of the world was in the dark about the full extent of the devastation, because Maria had destroyed the island’s main weather radar and almost all cell phone towers.
Far away on the U.S. West Coast, in Santa Cruz, Calif., oceanographer Olivia Cheriton watched satellite radar images of Maria passing over the instruments she and her U.S. Geological Survey team had anchored a few kilometers southwest of Puerto Rico. The instruments, placed offshore from the seaside town of La Parguera, were there to track pollution circulating around some of the island’s endangered corals.
More than half a year went by before she learned the improbable fate of those instruments: They had survived and had captured data revealing hurricane-related ocean dynamics that no scientist had ever recorded.
The wind-driven coastal currents interacted with the seafloor in a way that prevented Maria from drawing cold water from the depths of the sea up to the surface. The sea surface stayed as warm as bathwater. Heat is a hurricane’s fuel source, so a warmer sea surface leads to a more intense storm. As Cheriton figured out later, the phenomenon she stumbled upon likely played a role in maintaining Maria’s Category 4 status as it raked Puerto Rico for eight hours.
“There was absolutely no plan to capture the impact of a storm like Maria,” Cheriton says. “In fact, if we somehow could’ve known that a storm like that was going to occur, we wouldn’t have put hundreds of thousands of dollars’ worth of scientific instrumentation in the water.”
A storm’s path is guided by readily observable, large-scale atmospheric features such as trade winds and high-pressure zones. Its intensity, on the other hand, is driven by weather events inside the hurricane and wave action deep below the ocean’s surface. The findings by Cheriton and colleagues, published May 2021 in Science Advances, help explain why hurricanes often get stronger before making landfall and can therefore help forecasters make more accurate predictions.
Reef pollution
Cheriton’s original research objective was to figure out how sea currents transport polluted sediments from Guánica Bay — where the Lajas Valley drains into the Caribbean Sea — to the pristine marine ecosystems 10 kilometers west in La Parguera Natural Reserve, famous for its bioluminescent waters.
Endangered elkhorn and mountainous star corals, called “the poster children of Caribbean reef decline” by marine geologist Clark Sherman, live near shore in some of the world’s highest recorded concentrations of now-banned industrial chemicals. Those polychlorinated biphenyls, or PCBs, hinder coral reproduction, growth, feeding and defensive responses, says Sherman, of the University of Puerto Rico–Mayagüez.
Half of corals in the Caribbean have died since monitoring began in the 1970s, and pollution is a major cause, according to an April 2020 study in Science Advances. Of particular interest to Cheriton, Sherman and their colleagues was whether the pollution had reached deepwater, or mesophotic, reefs farther offshore, which could be a refuge for coral species that were known to be dying in shallower areas.
The main artery for this pollution is the Rio Loco — which translates to “Crazy River.” It spews a toxic runoff of eroded sediments from the Lajas Valley’s dirt roads and coffee plantations into Guánica Bay, which supports a vibrant fishing community. Other possible contributors to the pollution — oil spills, a fertilizer plant, sewage and now-defunct sugar mills — are the subject of investigations by public health researchers and the U.S. Environmental Protection Agency.
In June 2017, the team convened in La Parguera to install underwater sensors to measure and track the currents in this threatened marine environment. From Sherman’s lab on a tiny islet overrun with iguanas the size of house cats, he and Cheriton, along with team leader and USGS research geologist Curt Storlazzi and USGS physical scientist Joshua Logan, launched a boat into choppy seas.
At six sites near shore, Storlazzi, Sherman and Logan dove to the seafloor and used epoxy to anchor pressure gauges and batonlike current meters. Together the instruments measured hourly temperature, wave height and current speed. The team then moved farther offshore where the steep island shelf drops off at a 45-degree angle to a depth of 60 meters, but the heavy ocean chop scuttled their efforts to install instruments there.
For help working in the difficult conditions, Sherman enlisted two expert divers for a second attempt: Carlo, the geologist and diving safety officer, and marine scientist Evan Tuohy, both of the University of Puerto Rico–Mayagüez. The two were able to install the most important and largest piece, a hydroacoustic instrument comprising several drums fastened to a metal grid, which tracked the direction and speed of currents every minute using pulsating sound waves. A canister containing temperature and salinity sensors took readings every two minutes. Above this equipment, an electric thermometer extended to within 12 meters of the surface, registering temperature every five meters vertically every few seconds.
Working in concert, the instruments gave a high-resolution, seafloor-to-surface snapshot of the ocean’s hydrodynamics on a near-continuous basis. The equipment had to sit level on the sloping seafloor so as not to skew the measurements and remain firmly in place. Little did the researchers know that the instruments would soon be battered by one of the most destructive storms in history.
Becoming Maria
The word hurricane derives from the Caribbean Taino people’s Huricán, god of evil. Some of the strongest of these Atlantic tropical cyclones begin where scorching winds from the Sahara clash with moist subtropical air over the island nation of Cape Verde off western Africa. The worst of these atmospheric disturbances create severe thunderstorms with giant cumulonimbus clouds that flatten out against the stratosphere. Propelled by the Earth’s rotation, they begin to circle counterclockwise around each other — a phenomenon known as the Coriolis effect.
Weather conditions that summer had already spawned two monster hurricanes: Harvey and Irma. By late September, the extremely warm sea surface — 29º Celsius or hotter in some places — gave up its heat energy by way of evaporation into Maria’s rushing winds. All hurricanes begin as an area of low pressure, which in turn sucks in more wind, accelerating the rise of hot air, or convection. Countervailing winds known as shear can sometimes topple the cone of moist air spiraling upward. But that didn’t happen, so Maria continued to grow in size and intensity.
Meteorologists hoped that Maria would lose force as it moved across the Caribbean, weakened by the wake of cooler water Irma had churned up two weeks earlier. Instead, Maria tracked south, steaming toward the eastern Caribbean island of Dominica. Within 15 hours of making landfall, its maximum sustained wind speed doubled, reaching a house-leveling 260 kilometers per hour. That doubling intensified the storm from a milder (still dangerous) Category 1 to a strong Category 5.
NOAA’s computer forecasting models did not anticipate such rapid intensification. Irma had also raged with unforeseen intensity.
After striking Dominica hard, Maria’s eyewall broke down, replaced by an outer band of whipping thunderstorms. This slightly weakened Maria to 250 kilometers per hour before it hit Puerto Rico, while expanding the diameter of the storm’s eyewall — the area of strong winds and heaviest precipitation — to 52 kilometers. That’s close to the width of the island.
It’s still not fully understood why Maria had suddenly gone berserk. Various theories point to the influence of hot towers — convective bursts of heat energy from thunderclouds that punch up into the stratosphere — or deep warm pools, buoyant freshwater eddies spilling out of the Amazon and Orinoco rivers into the Atlantic, where currents carry these pockets of hurricane-fueling heat to the Gulf of Mexico and the Caribbean Sea.
But even though these smaller-scale events may have a big impact on intensity, they aren’t fully accounted for in weather models, says Hua Leighton, a scientist at the National Oceanic and Atmospheric Administration’s hurricane research division and the University of Miami’s Cooperative Institute for Marine and Atmospheric Studies. Leighton develops forecasting models and investigates rapid intensification of hurricanes.
“We cannot measure everything in the atmosphere,” Leighton says.
Without accurate data on all the factors that drive hurricane intensity, computer models can’t easily predict when the catalyzing events will occur, she says. Nor can models account for everything that happens inside the ocean during a hurricane. They don’t have the data.
Positioning instruments just before a hurricane hits is a major challenge. But NOAA is making progress. It has launched a new generation of hurricane weather buoys in the western North Atlantic and remote control surface sensors called Saildrones that examine the air-sea interface between hurricanes and the ocean (SN: 6/8/19, p. 24).
Underwater, NOAA uses other drones, or gliders, to profile the vast areas regularly traversed by tropical storms. These gliders collected 13,200 temperature and salinity readings in 2020. By contrast, the instruments that the team set in Puerto Rico’s waters in 2017 collected over 250 million data points, including current velocity and direction — a rare and especially valuable glimpse of hurricane-induced ocean dynamics at a single location.
A different view
After the storm passed, Storlazzi was sure the hurricane had destroyed his instruments. They weren’t designed to take that kind of punishment. The devices generally work in much calmer conditions, not the massive swells generated by Maria, which could increase water pressure to a level that would almost certainly crush instrument sensors.
But remarkably, the instruments were battered but not lost. Sherman, Carlo and Touhy retrieved them after Maria passed and put them in crates awaiting the research group’s return.
When Storlazzi and USGS oceanographer Kurt Rosenberger pried open the instrument casings in January 2018, no water gushed out. Good sign. The electronics appeared intact. And the lithium batteries had powered the rapid-fire sampling enterprise for the entire six-month duration. The researchers quickly downloaded a flood of data, backed it up and started transmitting it to Cheriton, who began sending back plots and graphs of what the readings showed.
Floodwaters from the massive rains brought by Maria had pushed a whole lot of polluted sediment to the reefs outside Guánica Bay, spiking PCB concentrations and threatening coral health. As of a few months after the storm, the pollution hadn’t reached the deeper reefs.
Then the researchers realized that their data told another story: what happens underwater during a massive hurricane. They presumed that other researchers had previously captured a profile of the churning ocean depths beneath a hurricane at the edge of a tropical island.
Remarkably, that was not the case.
“Nobody’s even measured this, let alone reported it in any published literature,” Cheriton says. The team began to explore the hurricane data not knowing where it might lead.
“What am I looking at here?” Cheriton kept asking herself as she plotted and analyzed temperature, current velocity and salinity values using computer algorithms. The temperature gradient that showed the ocean’s internal or underwater waves was different than anything she’d seen before.
During the hurricane, the top 20 meters of the Caribbean Sea had consistently remained at or above 26º C, a few degrees warmer than the layers beneath. But the surface waters should have been cooled if, as expected, Maria’s winds had acted like a big spoon, mixing the warm surface with cold water stirred up from the seafloor 50 to 80 meters below. Normally, the cooler surface temperature restricts the heat supply, weakening the hurricane. But the cold water wasn’t reaching the surface.
To try to make sense of what she was seeing, Cheriton imagined herself inside the data, in a protective bubble on the seafloor with the instruments as Maria swept over. Storlazzi worked alongside her analyzing the data, but focused on the sediments circulating around the coral reefs.
Cheriton was listening to “An Awesome Wave” by indie-pop band Alt-J and getting goosebumps while the data swirled before them. Drawing on instincts from her undergraduate astronomy training, she focused her mind’s eye on a constellation of data overhead and told Storlazzi to do the same.
“Look up Curt!” she said.
Up at the crest of the island shelf, where the seafloor drops off, the current velocity data revealed a broad stream of water gushing from the shore at almost 1 meter per second, as if from a fire hose. Several hours before Maria arrived, the wind-driven current had reversed direction and was now moving an order of magnitude faster. The rushing surface water thus became a barrier, trapping the cold water beneath it.
As a result, the surface stayed warm, increasing the force of the hurricane. The cooler layers below then started to pile up vertically into distinct layers, one on top of the other, beneath the gushing waters above.
Cheriton calculated that with the fire hose phenomenon the contribution from coastal waters in this area to Maria’s intensity was, on average, 65 percent greater, compared with what it would have been otherwise.
Oceanographer Travis Miles of Rutgers University in New Brunswick, N.J., who was not involved in the research, calls Cheriton and the team’s work a “frontier study” that draws researchers’ attention to near-shore processes. Miles can relate to Cheriton and her team’s accidental hurricane discovery from personal experience: When his water quality–sampling gliders wandered into Hurricane Irene’s path in 2011, they revealed that the ocean off the Jersey Shore had cooled in front of the storm. Irene’s onshore winds had induced seawater mixing across the broad continental shelf and lowered sea surface temperatures.
The Puerto Rico data show that offshore winds over a steep island shelf produced the opposite effect and should help researchers better understand storm-induced mixing of coastal areas, says NOAA senior scientist Hyun-Sook Kim, who was not involved in the research. It can help with identifying deficiencies in the computer models she relies on when providing guidance to storm-tracking meteorologists at the National Hurricane Center in Miami and the Joint Typhoon Warning Center in Hawaii.
And the unexpected findings also could help scientists get a better handle on coral reefs and the role they play in protecting coastlines. “The more we study the ocean, especially close to the coast,” Carlo says, “the more we can improve conditions for the coral and the people living on the island.”